A report on the DECHEMA conference “Organotypic Tissue Culture for Substance Evaluation” held in Potsdam, Germany, September, 2009
by Steve Dickman, CEO, CBT Advisors
CONFERENCE PROGRAM LINK http://events.dechema.de/Tagungen/Organotypic_Tissue_Culture_for_Substance_Evaluation-p-124158/Programme-p-124160.html
High-tech tissue culture models – loaded with multiple cell types on nano-optimized substrates, vascularized and even with access to reservoirs of stem cells – are about to become a new reality in preclinical testing, both regulatory and especially non-regulatory. Even though these complex models today account for a very small percentage of all preclinical testing models, a combination of factors will, in my view, drive more of these models to market in the relatively short time frame of three to five years. Factors that justify this aggressive time frame include:
- Regulatory pressure from legislation such as the European REACH;
- Demonstrated success of multiple-cell-type models such as 3-d skin models; and
- Burgeoning academic pursuit of ever more realistic tissue culture models.
Add the cost savings likely to arrive via outsourcing as soon as these models are reduced to practice and we have the perfect recipe for the near-instant growth of an industry: just add defined culture medium.
REACHing for new regulations
External drivers for the acceleration in animal alternatives include both policies and end markets. On the policy side, EU legislation banning animal tests for acute toxicity has already taken effect, with a commensurate boost in the market for non-animal skin tests especially for cosmetics. But with two laws, the European Union has put into play a much larger market. The so-called REACH legislation, agreed in 2006, demanded retrospective testing of all chemicals on the market. (REACH stands for Registration, Evaluation, Authorisation and Restriction of Chemical Substances.) And the 2003 amendment to the 1976 EU cosmetics directive, which eliminates all testing of cosmetic ingredients on animals by 2013, puts enormous pressure on cosmetics and consumer products manufacturers to replace animal tests.
The impact of these laws, both direct and indirect, will be far-reaching. The cosmetics directive, for example, will apply both to products developed in Europe and to imported products marketed in Europe. And REACH has already ‘reached’ beyond Europe’s borders. “The European Union will hold test systems to a higher standard and this in turn will impact FDA,” said Lynn Allen-Hoffmann, CEO and CSO of the US-based skin company Stratatech Corporation (http://www.stratatechcorp.com/). “I think the US requirements will become more stringent both regarding sourcing of material [for in vitro tests] and for endpoint assays.”
Though the push to replace animal tests in cosmetics has been grabbing headlines (see “Toxicity testing gets a makeover,” Nature 461: 158 (2009)), pharmaceutical R&D represents the real market. One 2002 publication, cited by industry insiders as authoritative, put the percentage of animal tests performed for cosmetics testing at 0.3% (Schumann, R. The Seventh Amendment to the Cosmetics Directive: what does DG Enterprise want from ECVAM? ATLA 30, Supplement 2, 213-214 (2002)).
The drivers for pharmaceutical R&D to adopt alternative assays not only involve the desire to minimize use of animals; pharma predominantly wants to lower R&D costs while increasing R&D productivity. Toxicity testing places a huge burden on pharma R&D budgets without delivering a big return on investment. Tox testing costs pharmaceutical companies $1.5 billion annually, according to industry consultancy Select Biosciences (Select Biosciences Report on Toxicity Screening Markets, Feb. 2009), while 63% of drugs that fail during clinical development do so for reasons of safety.
Pharma thus has two problems to solve: improving the quality of non-regulatory testing, for example with truly predictive efficacy models, while also reducing the cost of toxicity assays and other regulatory tests at no loss in assay fidelity. These challenges will be met by the increasingly complex single-tissue and multi-tissue models under development. “In vitro companies are coming up like fungi,” said Bart De Wever, a serial entrepreneur in the skin testing space who was a senior manager at SkinEthic Laboratories, acquired by L’Oreal in 2006.
Skin testing shows the way
Skin testing is the successful example that will show the way to companies working on other organs. The first human skin models were developed for the purpose of tissue engineering. The scientific and product development work was successful: in the 1990s, companies like Advanced Tissue Sciences (ATS) and Organogenesis brought to market their skin products, both used for treatment of burns and of diabetic foot ulcers as well as for in vitro testing. ATS even had an IPO. However, the companies did not recognize the enormous challenge they faced in obtaining true market acceptance and reimbursement for products with a high cost of goods. By 2002, both of these first-generation companies were either bankrupt or had reorganized.

Stratatech 3-d skin model (http://www.stratatechcorp.com/science/index.php)
More recently, other skin model companies have found products. These companies have focused less on engineering tissues for clinical use and more on skin toxicity or skin corrosion testing. These include the French company EPISKIN (acquired by L’Oreal in 1997) and US-based MatTek (still private and independent) as well as SkinEthic (France), CellSystems (Germany), Phenion (Germany – now part of Henkel AG), StratiCell (Belgium) and US-based StrataTech. All of the skin models in current use are three-dimensional and some are able to recreate the multilayered, multi-cell-type qualities of actual human skin. “It is well documented in the scientific literature that the response of this tissue is much more predictive than the classical monolayer response,” said De Wever. Furthermore, these 3-d skin models have recently become the “gold standard” for topical substance evaluation, endorsed by the 30-nation OECD (Organisation for Economic Co-operation and Development).
The next big barrier to fall for skin models will be regulatory in nature. “Animal testing is still the regulatory gold standard,” said De Wever. “Many regulators do not seem to understand in vitro data. It has been (incorrectly!) explained to them that data obtained in in vitro models is ‘simpler’ and therefore less predictive,” no matter how complex the in vitro models become. This is bound to change, probably first in Europe and then elsewhere, under the growing pressure of the new regulatory regime imposed by REACH and the cosmetics legislation.
In the meantime, new science is laying the groundwork for tissue tests in many complex organs. Areas advancing quickly include nanomaterials and surface science; the addition of rare cells; and adding vasculature to multi-cell-type models.
Nano: the next frontier
Biology is ultimately physics. Physics underlies every biological process: cell division, cell growth, mutation, selection and especially cells’ interaction with their environment. Therefore it is not surprising that physical factors are increasingly important as tissue culture systems increase in complexity.

Cells move right to left, nudged by the ratchet-like pattern of their substrate (Mahmud et al., Nature Physics 5, 606 - 612 (2009))
In his conference-opening lecture in Potsdam, Prasad Shastri of the University of Freiburg described a paper in Nature Physics (Nature Physics 5, 606 – 612 (2009)) by the Grzybowski group at Northwestern University in which “asymmetric micro-geometries” such as micro-ratchets can be used to control otherwise random motion of cells. Imposing order on cells through passive means such as the nano-architecture of their substrates is an extremely promising area. Shastri’s own work has shown that the “nanoroughness” of a cell’s environment impacts the timing of cell cycle events such as DNA synthesis. The changes observed are the same as when growth factors are applied. “Environment is a clue we had previously ignored,” he says.
Sculpting the right nanoenvironment is even more important for drug and nutrient delivery. Monika Schaefer-Korting from the Free University of Berlin showed that uptake of opioids through the skin could be greatly enhanced beyond the standard 1-3% by loading them onto lipid nanoparticles. Michael Buchmeiser of the Leibniz Institute of Surface Modification in Leipzig showed early studies on how calcium carbonate and hydroxyapatite nanostructures could be created that delivered ideal amounts of calcium to cells growing nearby.
Adding rare cells
Another frontier rapidly being mastered by tissue culture pioneers is the addition of rare cell types. “Skin consists of more cell types than keratinocytes and fibroblasts,” said de Wever. So skin modelers such as Heike Walles (née Mertsching) are adding adipocytes. These cells “store substances over a long period. If you want to study [skin] over the long term, you need adipocytes,” said Walles, who is Chair of Tissue Engineering and Regenerative Medicine at the University of Würzburg in Germany, in her conference lecture.
In a similar way, Dr. Hemant Kocher of the Tumour Biology Laboratory of the Barts & the London School of Medicine showed compellingly in his lecture that novel in vitro organotypic pancreatic tumor models could become extremely predictive if a single rare cell population – the stellate cells – were added to cultures of pancreatic cells (Am J Pathol 175(2):636-48 (2009)). The underlying logic is striking: stellate cells, which were first isolated in 1998 by Apte et al. from rat pancreas (Gut 43:128-133(1998)), are stromal cells and are vital for stromal reaction in pancreatic cancer. They serve as storehouses of, among other substances, vitamin A. Perhaps, Kocher reasoned, they are also beacons instructing neighboring cells to increase or decrease their proliferation or metabolic activity. Sure enough, Kocher showed that modifying stellate cell behavior can be used to alter tumor behavior. “Using this pancreatic cancer model, we can dissect out the crosstalk between tumor & stroma … and directly target the stroma,” said Kocher. This model provides a useful assay for testing drug candidates and might lead to therapies that previously would not have been identified in a cancer where virtually all traditional approaches have failed.
Adding vasculature
“Single cells are destined to die.” That statement, made by Helmut Augustin of the German Cancer Research Center in Heidelberg in his lecture, neatly captures the necessity for seeding multiple cells into tissue models. But multiple cells and even multiple cell types are not enough. One must incorporate functional structures like blood vessels or even nerves if tissue models are ever to be truly life-like. This puts endothelial cells in the spotlight. The worldwide leader in vascularized tissue models is Heike Walles of the University of Würzburg. Walles’ tissue models range from skin, intestine and liver to tumor and trachea.
Vascularizing tissue grown in culture allows Walles and her colleagues to overcome the size limitation that otherwise plagues tissue engineers. “In liver and other organs with high metabolic activity,” she said, “we and others could show that cells more than 0.8mm from a blood vessel do not get enough oxygen.” Choosing pig jejunum was a choice inspired by necessity. Artificial scaffolds were limited to a vessel diameter of 3mm. “There was no way to generate a complex implant with that method,” recalled Walles. But by isolating the jejunum of a pig, the middle portion of its small intestine, and eliminating the porcine cells, Walles was able to seed endothelial cells into the remaining tubular structures and create functioning networks of blood vessels. The tracheal tissue she and her team crafted on pig jejunum from autologous human cells have even been successfully applied in the clinic, plugging holes in the trachea that resulted from trauma or surgery.
Multicellular models: from stem cells to waste disposal
The most ambitious tissue models described at the conference – for either substance testing or for tissue engineering – combine all the elements described above: they are vascularized, contain multiple cell types including rare cell types and are constructed in physical environments conducive to controlled cell proliferation. One site where all these technologies are coming together is the Fraunhofer Institute for Material and Beam Technology (IWS), Dresden, Germany, coupled with the Department of Medical Biotechnology at the Technical University of Berlin. In Dresden, a group led by Frank Sonntag is working toward a very ambitious systemic toxicity platform using a “chip-based multi-organoid culture system for research and substance testing.” They are simultaneously pursuing three tissues for tox testing: liver, brain cortex and bone marrow.

Above, a model for the ideal multi-tissue micro-bioreactor; below, a schematic of the prototype microbioreactor of Frank Sonntag at the IWS in Dresden, which incorporates three organ growth segments, a stem cell niche cavity, three behavior sensors, three waste reservoirs and interconnecting channels. (Images courtesy Frank Sonntag)
Sonntag defined the micro-organoid as “the in vitro equivalent to the smallest functionally self-reliant unit of a human organ, such as liver lobuli, neuronal layers of the cortex, specula in the bone marrow, nephrons in the kidney or the alveoli in lung.” By focusing on the needs of the functional units, for example in constructing the physical shape and scale of their microenvironment, Sonntag and his team hope to achieve the most lifelike responses yet. And by making sure to include multiple components including multiple cell types, vasculature and stem cells, he hopes to greatly extend the useful life of his “micro-bioreactors.”
Sonntag’s work echoes that of Sangeeta Bhatia, a professor at the Massachusetts Institute of Technology (MIT) in Cambridge (who did not speak at the conference) whose group has made important progress toward weeks-long cultures of primary human hepatocytes, a step that might prove very useful for in vitro drug testing. However, there is one important difference: Bhatia, who has founded a company, Hepregen, is focusing for the moment on a single tissue, the liver, although the principles she discovers may someday be applicable to culturing other organs.
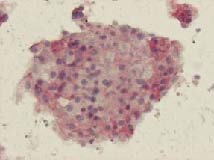
Micro-organoid formation out of diverse lymph node cells in agarose matrix (Image courtesy Probiogen)
Sonntag’s mention of micro-organoids also echoes the commercial-stage work on an “artificial lymph node” developed by Berlin-based company ProBioGen. Here too, the principle is to create the smallest possible functional unit, this time from multiple cell types via a process that Christoph Giese, the Head of ProBioGen’s department of Cell and Tissue Services, in his talk at the conference called “in vitro controlled organogenesis.” Cells are prepared in complex co-culture (that is, with multiple cell types), perfused with the optimal defined culture medium in a 3-d macroenvironment, said Giese, then harvested and seeded onto the bioreactor platform, which includes the stromal cells also found by others to be so important. Remarkably, the system demonstrates appropriate “immune responses”, both T-cell and B-cell-based, to antigens such as viral proteins presented in the medium. The system is already in use for testing substances both in normal and disease states.
As important as it is that the micro-scale, multi-tissue cultures described here be lifelike, viable and predictive, it is equally important if not more important that they also be manufacturable, scalable and ultimately available at multiple price points. It might seem early in the game to be thinking about the path to commercial success but in the end that will be the ultimate proof.
How close are these techniques to widespread application? It took 3-d skin models close to ten years, roughly from 1997 to 2007, to achieve widespread adoption. The efforts to combine multiple tissue types on a single platform – such as Sonntag’s – have begun in the past two or three years and are anticipating significant milestones including full proof-of-concept in the 2011 time frame. But given the pace of development – with parallel efforts springing up in Europe and the United States, significant industry interest and experienced entrepreneurs who have created companies in the past, it is easy to believe that the pace might be even quicker.